Solar power. It comes in two primary flavors: photovoltaics (PV) and concentrated solar power (CSP). The latter is easy. I decided to do solar power this week, and go back to the dams next week. Big picture: CSP is a bridge technology at best; an investment in most places is little more than a show that the investor is serious about green tech. Moreover, not all places are created equal to invest in solar power. Many of the places that offer the best incentives to have solar power (NJ, MA, Germany) are far from the best places to have solar power.
So this time: insolation, what it means, where it happens. And CSP. PV comes later. Cause it involves quantum mechanics.
So, first, solar insolation map, AKA "Where is the sun shining all the time" map.
Who is not surprised that Alaska is awful for solar power? But check out MA and NJ. Why are they giving tax breaks to install solar cells? Easy answer. To drive the technology forward. Solar panels are really useful in places without any other power source. Like small villages in Africa and other depopulated places. California also has big incentives to build solar, and at least that makes sense, yes?
What determines how much insolation a place gets? Well, you need sun to have solar power. The sun doesn't come out to party at night, so no solar power. A huge one is how much atmosphere the sunlight has to pass through on the way to the the solar panel. More atmosphere means more absorption and dispersing of sunlight (the atmosphere reflects, absorbs, and spreads out sunlight). So higher elevation, like mountains, helps. Less atmosphere. On a related note, the latitude is also very important. Far northern places don't get as much sun annually (Canada, Alaska). Finally cloud and moisture make a huge difference. If there are clouds or moisture in general, sunlight is blocked. This explains most of the east coast of the US, as well as why Nevada, a giant desert, has great insolation. It has a high elevation, and no moisture to make clouds or block sunlight.
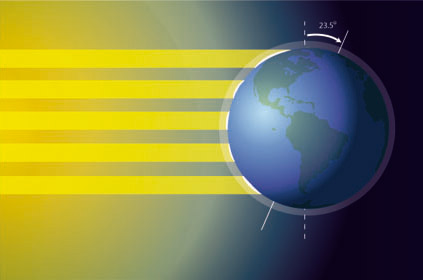
The equivalent amount of sunlight hitting the earth at a high latitude spills out over a larger area. In other words, there amount of energy per area is lower. link
CSP is easy. There are a bunch of mirrors, either flat or parabolic (to focus the light even more intensely), and they reflect light to a single point. It produces heat and and then that heat is used to make steam and drive a turbine, just like the basic thermal power plants we have discussed. The heat is typically stored in molten salt, cause it can store a whole lot of energy before it rises a degree in temperature (kind of like water). The heat from this molten salt is slowly released to make that steam for the thermal part.
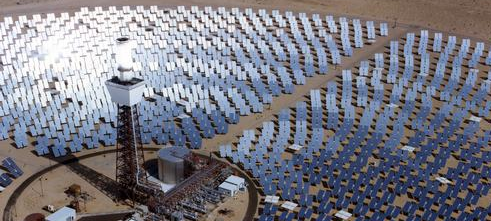
CSP in action. Lots of light reflected to a single point that then gets very hot. link.
Given that some places on Earth receive upwards of 500W/m directly to the surface (assuming no clouds, no pollution, and daytime), a CSP plant that is 500m*500m could produce 125MW of power. Sounds great, right? 'Cept we know from basic thermodynamics that a thermal power plant that this thing is likely going to be 30% efficient. So something with a quarter of a square kilometer footprint might produce 40MW of power.
So why don't we use this? First, the depiction aboveĀ is too rosy a picture. CSP is not all that efficient, because if you look at the picture above, you see that not all the area is used for gathering light. There are plenty of empty spaces. Moreover, the transfer of heat from the salt to water is not very efficient. Cause the high temperature and low temperature of the Carnot cycle are closer together (review the thermal power plant post for a review of Carnot efficiencies for all heat engines). Finally, this stuff is expensive. It is easily 2x as expensive as almost any other power technology (other than PV). It requires water to clean the mirrors and has other maintenance costs, the mirrors themselves are quite expensive, and the entire design is expensive. And, if you want to harness the power of the sun, there are better alternatives. Like PV.
As you can tell, I don't have a very high opinion of CSP. Why is that? Take a look at this guy again:
U.S. average levelized costs (2011 $/megawatthour) for plants entering service in 2018 | ||||||
---|---|---|---|---|---|---|
Plant type | Capacity factor (%) | Levelized capital cost | Fixed O&M | Variable O&M (including fuel) | Transmission investment | Total system levelized cost |
Dispatchable Technologies | ||||||
Conventional Coal | 85 | 65.7 | 4.1 | 29.2 | 1.2 | 100.1 |
Advanced Coal | 85 | 84.4 | 6.8 | 30.7 | 1.2 | 123.0 |
Advanced Coal with CCS | 85 | 88.4 | 8.8 | 37.2 | 1.2 | 135.5 |
Natural Gas-fired | ||||||
Conventional Combined Cycle | 87 | 15.8 | 1.7 | 48.4 | 1.2 | 67.1 |
Advanced Combined Cycle | 87 | 17.4 | 2.0 | 45.0 | 1.2 | 65.6 |
Advanced CC with CCS | 87 | 34.0 | 4.1 | 54.1 | 1.2 | 93.4 |
Conventional Combustion Turbine | 30 | 44.2 | 2.7 | 80.0 | 3.4 | 130.3 |
Advanced Combustion Turbine | 30 | 30.4 | 2.6 | 68.2 | 3.4 | 104.6 |
Advanced Nuclear | 90 | 83.4 | 11.6 | 12.3 | 1.1 | 108.4 |
Geothermal | 92 | 76.2 | 12.0 | 0.0 | 1.4 | 89.6 |
Biomass | 83 | 53.2 | 14.3 | 42.3 | 1.2 | 111.0 |
Non-Dispatchable Technologies | ||||||
Wind | 34 | 70.3 | 13.1 | 0.0 | 3.2 | 86.6 |
Wind-Offshore | 37 | 193.4 | 22.4 | 0.0 | 5.7 | 221.5 |
Solar PV1 | 25 | 130.4 | 9.9 | 0.0 | 4.0 | 144.3 |
Solar Thermal | 20 | 214.2 | 41.4 | 0.0 | 5.9 | 261.5 |
Hydro2 | 52 | 78.1 | 4.1 | 6.1 | 2.0 | 90.3 |
Pingback: Power Grid | Jason Munster's Energy and Environment Blog