Electric Cars. Are they really all they are hyped up to be? The short answer: hell yeah. These things are sweet. I want to get my hands on one right now.
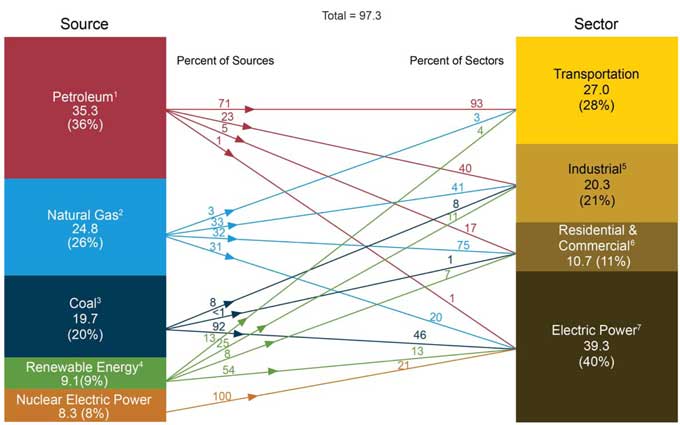
Energy flows in the US. Transportation accounts for 28% of all energy use, primarily from burning petroleum.
35% of US energy consumption is in transportation. Transportation requires that the energy source be within the vehicle (unless you are in South Korea, where the energy source is induction and is beneath the road. Pretty badass, if you ask me). Batteries currently weigh a lot, don't have nearly as much energy per pound as gasoline, and require a long time to charge. But if we could replace a huge percent of this with more efficient electric cars, it would go a long ways towards arresting GHG emissions.
120 million Americans commute to work by car. The average person lives fewer than 20 miles from work. Substantially all of them commute alone. The Nissan Leaf gets 75 miles before it needs to be recharged. The Tesla model S goes about 275 miles. No matter what the source of energy for an electric car, it produces less CO2 than a normal car.
How does an electric car produce less CO2 than a gas one? No matter the source of the electricity, even if it is an old inefficient coal plant, the conversion efficiency of an electric car will result in lower CO2 emissions per mile than a gas powered car. The EPA estimates that the Nissan Leaf gets an estimated 125mpg using CO2 equivalent of gasoline. The recent fleet average for the US is about 30mpg for passenger cars. So electric cars emit only 25% the CO2 of your average normal car. Lets be generous and say they emit 40% the CO2 of your best gasoline powered cars.
Commuters would make a very significant difference in emissions if they changed over to electric cars.
Power
Most Americans base the acceleration needs of their car on the idea that they someday need to accelerate down on onramp to get to 65mph on the highway. The amount of power a car has is typically listed as horsepower (hp). This is a terrible measure. The real measure of power of a vehicle is Peak Torque.
Allow me to explain this concept. Roughly speaking, torque is the force going into a rotation of an object. It makes sense to use torque to describe cars, cause they have rotation parts. Think of it as the amount of energy going into the car from the tires rotating on the road.
(yes, that is a cross product) where r is the radius, or distance from the center of rotation, and F is the force. For the most part, the torque of a vehicle is entirely determined by its engine. It directly translates to how fast you can accelerate. More torque yields less time from zero to 60.
Let's compare some examples. First, my favorite. My bike, a Kawasaki VN750, vs. a Hayabusa (fastest production bike in the world) and an electric bike from Zero Motorcycles, the Zero DS. The electric motorcycle gets up to the equivalent of 400mpg. Compare to a normal bike of around 40-50mpg.
VN750 | Hayabusa | DS-electric | |
Style | Cruiser | Sport | Sort of cruiser |
Weight | 500 lbs | 563 lbs | 400 lbs |
Torque (ft-lbs) | 47 | 99.6 |
69 |
Before comparing, let's talk about peak torque. Peak torque is the maximum torque an engine can put out. For a gasoline engine, it is pretty much right before it redlines. So the numbers of 47 and 99.6 you see for the first two bikes means that it is the best they can do. You can think of an electric motor as pretty much always putting out peak torque. In other words, the hayabusa has to jump up to 6500rpm before it can be at full power, then it shifts up a gear, and drops back down out of its full power range. The electric bike doesn't shift gears, either.
Let's look at the Hayabusa power band to illustrate this difference.
As you can see, the torque output of a gas engine changes with RPM. You will notice the Kawasaki ZX-14 has more max torque, but less torque at the lower end. This is one of the main reasons the 'Busa is considered faster. It comes off the line far faster than other bikes, cause it has higher starting torque. Compare this to an electric engine, which has max torque from 0 RPMs up. You probably see my point about how sweet electric engines are.
So now we can compare electric vs gas based on torque. The electric motorcycle trounces my motorcycle all the time. In the first few moments, it will likely nearly match the Hayabusa. In fact, until the 'Busa hits 3000 RPM, the electric bike won't look too shabby. Why? First, cause it has the same torque as the 'Busa up til the Busa hits 3000rpm. Second, cause it weighs 150 lbs. less. In short, a smaller electric bike kicks butt. (note that the electric bike doesn't have super wide tires to accommodate all its power, so it might slide around a bit when you hammer down).
Where does the electric bike fall short? Range. This bad boy will only go 75 miles on the highway between charges. Funny enough, it'll go 125 in the city. This is all owed to wind resistance. Back to the point, you can't refill this guy as easily. You need to plug it in. It takes a while to recharge. You can just fill up a motorcycle and go on your way. This bike is pretty much for commuting or visiting friends in nearby cities.
(*2017 update! New electric motorcycles are capable of going 200 miles. Then they have to recharge for a long time. But 200 miles is a long trip).
Also, let's admit it, both my bike and the 'Busa are sexier.
The cars.
Audi A5 | Nissan Leaf | Tesla S | |
Cost | $38k | $21.3k | 62,000 |
MPG | 22 | 102 | 90 |
Peak Torque | 258 | 210 | 443 |
Weight (lbs) | 3549 | 3354 | 4650 |
Range (miles) | unlimited | 75 | 275 |
As you can see, if you are commuting the Nissan leaf makes a lot more sense in every possible way. It costs less to buy than most cars, it has the acceleration potential of a high-end Audi A-5, and the range you need to get to work and back. This beast will accelerate onto the highway just fine. Also, there is the whole power band thing. These electric cars have peak torque all the time.
Overview
Electric vehicles. These puppies accelerate as fast or faster than most vehicles in their class. The shorter range ones are less expensive to buy than most cars, and the cost of making them move is lower. Oh, and they save the environment relative to normal cars.
Whats the drawback? Range anxiety: the other event people think of when they don't want an electric car. Visiting Grandma. People want to buy one vehicle that can do everything they want. There is also the concern of "what if I am in an emergency and really need a car that can drive far right away?!?" How many times has that happened to you in your life? For me, the answer is 0. Any family emergency I had, I took a plane or a bus to.
Most families still own two cars. At least one should be electric. Here's an idea: get one gasoline car to visit grandma when you need, and then get an electric for your commute. Here's another idea: get an electric car or two, and rent a car when you need to go visit Grandma. You will save money either way.
The ridiculousness that is trucks and SUVs? Get a subaru with a roof rack, and rent the trucks and SUVs otherwise. Why the heck are you in a vehicle getting 12 miles to the gallon when gas is expensive, and when burning that gas helps wreck the environment? You, person who commutes to work in a pickup, are a selfish person.
Grid Stabilization
In the Solar and Wind articles, we read that these technologies produce intermittent power. In other words, they can't provide power on demand or at night. Imagine, if you will, that those 120,000,000 commuters all had electric cars. And that they all had excess batter capacity. They could charge up while the wind was blowing and the sun was shining, and discharge while the sun was sleeping and while the wind was lazy. Suddenly part of the problem with wind and solar has some help. This is a huge topic, though, and I won't go farther into it.
Shortest version:
Get an electric car for your commute.
Hokay, that is all for now. I will edit this as I get comments. Thanks for reading!
Jason Munster